Agroecology 4 min
Dominant and recessive gene expression, chapter One: evolutionary hypotheses
The dominant and recessive relationship between alleles offers a selective advantage in the self-incompatibility mechanism of certain flowering plants. This model enabled researchers to formulate hypotheses regarding the molecular mechanisms of genetic dominance.
Published on 17 December 2014
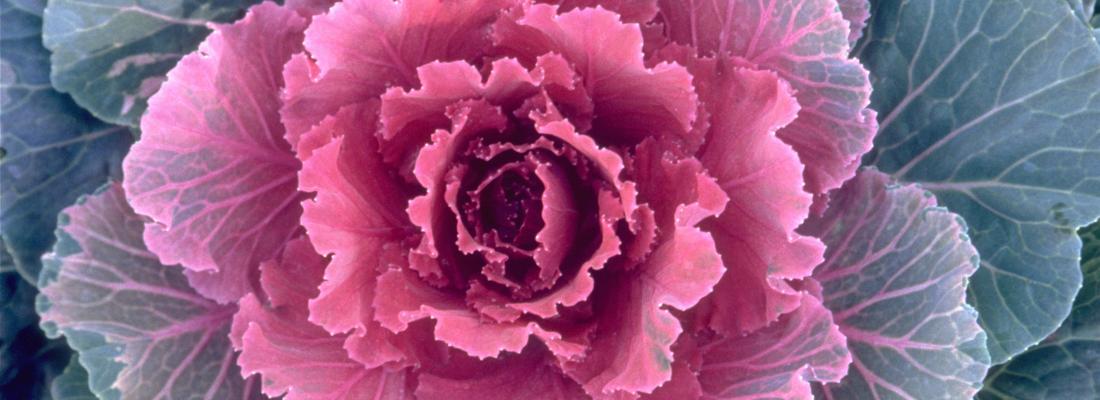
It all started with a controversy
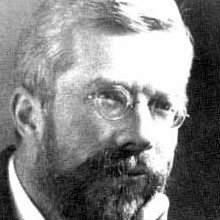
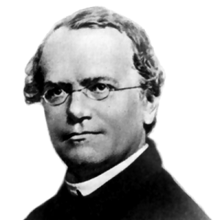
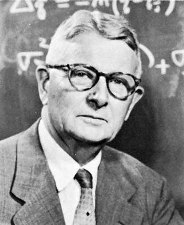
The story of genetic dominance starts with a controversy – and one of the most hotly-debated ones in the history of evolutionary biology at that – that pitted two of the major pioneers in the field, Ronald Aylmer Fisher and Sewall Green Wright against each other in the 1930s. Genetic dominance was one of the earliest genetic phenomena described by Gregor Mendel. The theory was that at a given gene locus of a heterozygous individual, one of the two homologous alleles prevailed over the other and controlled the phenotype. Blood types offer a well-known example. A and B types are dominant over O types, which means that A/O heterozygous individuals express A blood type and not O. However, this does not explain what determines whether certain alleles are dominant and others recessive, or how the process works. Fisher believed that dominance was possibly a direct consequence of natural selection, with the best alleles being selected and becoming “dominant”. Wright, however, thought that the effects of natural selection were not strong enough to explain the phenomenon and hypothesised that it was linked to how genes functioned and an intrinsic gene characteristic – a characteristic that had yet to be discovered. History would finally prove him right nearly a century later.
Studying self-incompatibility in flowering plants: recognising the self to prevent inbreeding
The self-incompatibility mechanism offers a perfect model for studying dominant and recessive alleles. The mechanism exists in a number of hermaphroditic species, such as cabbage, lettuce and turnips, and developed over time to prevent plants from self-fertilizing (1). Plants have pollen and a pistil, which each carry a protein: a ligand for the former, a receptor for the latter, functioning similarly to a key and lock. This mechanism is what enables the plant to recognise the self and nonself. If the “key” from an organism is inserted into its own “lock”, reproduction is blocked. This process does not occur when the key and lock are identified as coming from different individuals.
There is huge amount of diversity of keys and locks. The keys are encoded by one gene and the locks by another, with both being located in a specific part of the plant’s genome: the self-incompatibility locus. There are a dozen different versions of each of these genes (i.e., alleles) which produce the many keys and locks.
Additionally, because the plant’s genome is diploid, it has two alleles of each gene. Each individual can therefore have two different keys. If these two keys were active, heterozygous individuals would have access to fewer mates than individuals with only one key. The purpose of allelic dominance is clearer: If one of the alleles is dominant and the other recessive, only one key is expressed in heterozygotes. Selection via this type of system favours diversity and gives rare phenotypes an advantage as they have access to a greater number of possible mates.
Diversity leads to more complex relationships between alleles
The self-incompatibility mechanism relies on a large number of alleles encoding the determinants for the pollen and pistil, as well as the dominant and recessive phenomenon that ensures that heterozygotes express a single determinant. However, another question arises: Because the diversity among existing alleles generates numerous combinations of homologous allelic pairs, how are dominant and recessive relationships managed within this “network”?
Scientists hypothesised that there were genetic elements unique to each allele that controlled these relationships of dominance and that could be qualified as “dominance modifiers”. These elements were characterised only very recently (see Chapter Two).
(1) Self-fertilization (fertilization between the pollen and pistil of a single individual) promotes homozygosity, which enables the expression of recessive deleterious mutations, a phenomenon known as inbreeding depression.
- Locus: in genetics, a locus is a specific, invariable position on a chromosome. Genes are located at loci.
- Allele: a gene’s alleles are the alternative forms of the gene, characterised by variations in the DNA sequence. Different alleles can produce different proteins, resulting in a different phenotypic trait.
- Diploid: a cell is diploid when its chromosomes are found in pairs (2n chromosomes). The concept is generally the opposite of haploid, which refers to cells with a single set of chromosomes (n chromosomes).
- Homologous: in a diploid organism, each pair of chromosomes is composed of “homologous chromosomes”, which means one copy was inherited from the male gamete (“father”) and the other from the female gamete (“mother”).
- Homozygous: an individual is homozygous for a gene when it carries the same allele of that gene at a specific locus.
- Heterozygous: an individual is heterozygous for a gene when it carries different alleles of this gene at a specific locus.
- Phenotype:the observable or measureable trait(s) of an individual.