Agroecology Reading time 4 min
Crossing over with Raphaël Mercier: the mechanics of meiosis
Raphaël Mercier and his research team have made several major discoveries that have greatly advanced our understanding of meiosis; more specifically, they have focused on crossing-over, a process during which genetic material is exchanged between chromosomes. Mercier’s work promises to revolutionize the field of plant breeding because it suggests it is possible to customize the degree of genetic recombination. We asked Mercier to answer three questions about his work.
Published on 17 March 2016
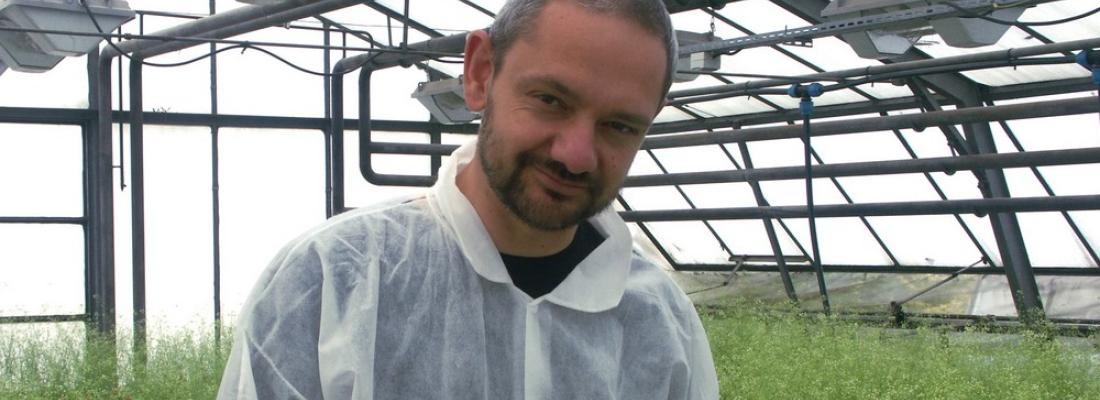
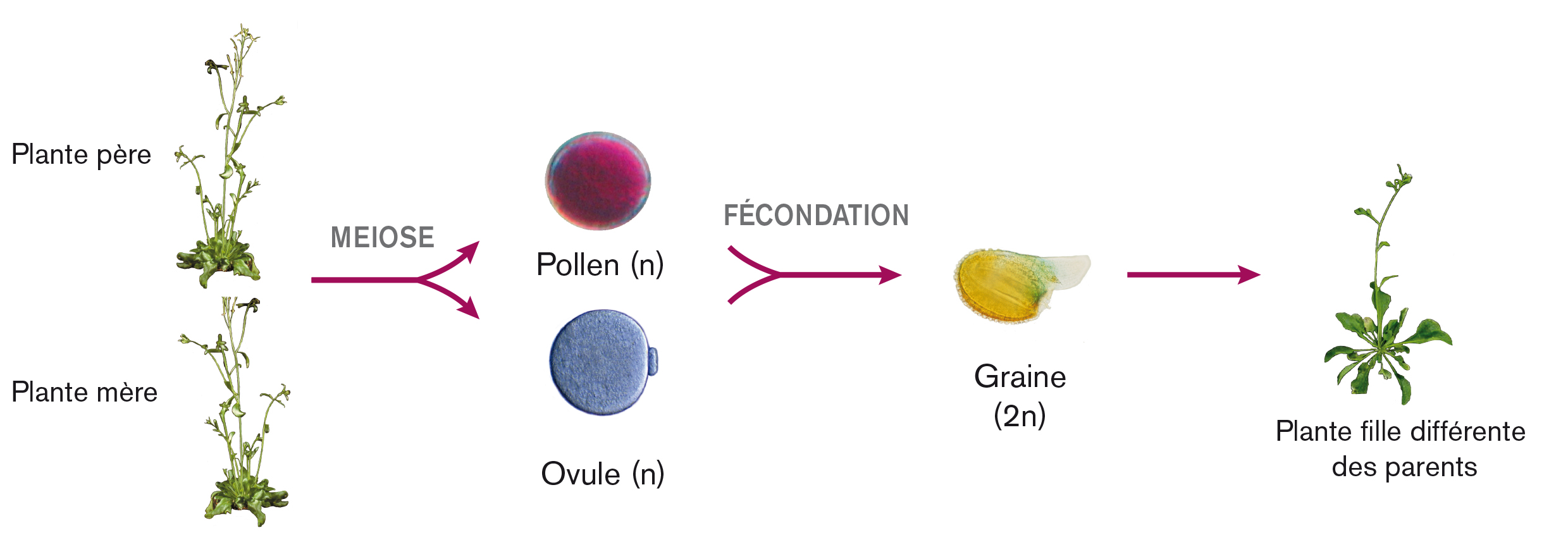
In species that reproduce sexually, offspring are genetically distinct from their parents because they inherit genetic material from both. Siblings are also distinct from one another. Such genetic diversity is the product of meiosis, a type of cellular division that creates reproductive cells from a parent cell. It occurs in both males and females. During meiosis, the chromosomes in the parent cell, which are organized in pairs (1), replicate. The genetic material is then separated as the cell divides once and then twice, forming a total of four reproductive cells. However, before they are separated, the paired chromosomes, also referred to as “homologous chromosomes,” can exchange segments of DNA via a mechanism called crossing-over. Since 2003, Raphaël Mercier and his research team have been studying meiosis and crossing-over, which both play a crucial role in generating genetic diversity within species.
What is the function of crossing-over?
Raphaël Mercier: Crossing-over has two main functions. The first is to increase genetic recombination. The second is to ensure that parental chromosomes are equitably distributed among the reproductive cells produced by meiosis. During crossing-over, homologous chromosomes are stuck together, which means that they will be properly positioned across from each other on the spindle apparatus, a web mostly made up of microtubules that segregates the pairs into two new daughter cells during the first meiotic division (2). Without crossing-over, the chromosomes would be distributed willy-nilly. We can see the importance of this process by looking at trisomy 21 (i.e., Down syndrome) in humans. In the absence of crossing-over, the two copies of chromosome 21 fail to segregate and end up in the same ovule. As a result, when the ovule is fertilized by a sperm cell, the embryo contains three copies of chromosome 21 instead of two (3).
Crossing-over is therefore very important. That said, you also do not want to overdo it! Across all species, there are an average of two to three crossing-over events per chromosome during meiosis (4). This number is very consistent and appears to be tightly constrained by several molecular mechanisms.
Slideshow: The stages of meiosis and the role of crossing-over in the segregation of homologous chromosomes among daughter cells:
Why so few crossing-over events?
R. Mercier: There are two hypotheses. The first relates to the mechanics: an overabundance of crossing-over events would prevent the homologous chromosomes from properly segregating. In our research, we have found limited evidence for this hypothesis because, for example, we have obtained mutants in whom meiosis is completely normal except that crossing-over events are nine times more frequent (5). The second hypothesis is genetic in nature: too many crossing-over events could disrupt advantageous gene combinations that have established themselves over evolutionary time. In this case, overzealous crossing-over could have long-term costs for the species.
Then why try to increase the frequency of crossing-over?
R. Mercier: Increasing the number of crossing-over events could lead to more genetic recombination and thus novel gene combinations, a desirable outcome in the context of plant breeding. Indeed, in Arabidopsis thaliana (6), we have been able to increase crossing-over frequencies up to nine times without negatively affecting meiosis. We have done so by inhibiting “anti-crossing-over” genes using targeted mutations. In collaboration with other INRA research teams, we are testing the effects of such mutations in other species, including peas, tomatoes, and rapeseed. The development of techniques that will allow us to modify entire genomes (7) will make it possible to apply our approach to numerous other species.
(1) In most species, individuals possess two copies of each chromosome. Such organisms are said to be diploid, and we use the notation 2n to indicate that their chromosomes exist in pairs. Individuals or cells with a single copy of each chromosome are haploid (n). Humans have 23 pairs of chromosomes, and the model plant species Arabidopsis thaliana has 5.
(2) See the slideshow.
(3) Chromosomal anomalies (e.g., aneuploidy) result in 30 to 40% of spontaneous abortions. This frequency is higher than people tend to realize.
(4) There are a few exceptions. For example, bees have a higher rate of crossing-over, as does the yeast Schizosaccharomyces pombe. In the latter, each chromosome experiences an average of 15 crossing-over events during meiosis.
(5) See sidebar 1.
(6) Arabidopsis thaliana: a flowering plant species in the Brassicaceae family that is an important model system in biological studies.
Crossing-over is essentially the exchange of DNA fragments between two chromosomes. For crossing-over to occur, it is essential to generate breaks in the chromosomes’ double-stranded DNA. These breaks trigger repair mechanisms; however, at most break sites, no crossing-over occurs and the strands remain unchanged. The number of breaks generated is therefore much higher than the number of crossing-over events (25 times higher in Arabidopsis).
Raphaël Mercier and his team have identified three genes that may limit the number of crossing-over events in the model species Arabidopsis thaliana. These genes are highly conserved across all species. Two of the genes, fancm and recq4, encode helicases, enzymes that play a generalized role in DNA repair. Mutations in these two genes are responsible for two genetic diseases: Fanconi anemia and Bloom syndrome. The third gene, figl1, is highly conserved in humans and codes for a protein that regulates the early stages of DNA repair.
A double mutation involving the fancm and recq4 genes increases crossing-over frequency by a factor of nine.
References:
-The fancm gene (encodes a helicase):
Crismani et al. 2012. Science 336(6088): 1588–1590. DOI: 10.1126/science.1220381. Read the press release.
-The recq4 gene (encodes a helicase):
Wu et al. 2003. Nature 426 (6968):870–874. DOI: 10.1038/nature02253.
-Double mutation involving the fancm and recq4 genes:
Séguéla-Arnaud et al. 2015. Proc Natl Acad Sci 112 (15):4713–4718. DOI: 10.1073/pnas.1423107112
-The figl1 gene (encodes fidgetin-like protein 1, an ATPase):
Girard et al. 2015. PLOS Genetics DOI: 10.1371/journal.pgen.1005369.
Raphaël Mercier and his team are also taking the opposite approach. They are attempting to put the brakes on genetic recombination mediated by crossing-over. The goal is to obtain seeds that are genetically identical to the mother plant, thus mimicking a rare natural process known as apomixis. Mercier comments, “If we are successful in our efforts, that is to say, if we can obtain a plant that produces seeds that are genetically identical to itself, then we will really be able to revolutionize the world of plant breeding. In particular, it would be great for hybrids to reproduce directly instead of having to constantly recreate them via crosses. It would remove a lot of constraints and allow us to more quickly and more economically produce hybrids that are better adapted to all sorts of environments.” At present, Mercier and colleagues are able to produce “clonal” seeds around 30% of the time in Arabidopsis, and they are currently trying out their techniques in different crop species.